Inorganic Nutrient Interaction in Mariculture Production
- Romi Novriadi
- Feb 18, 2016
- 9 min read
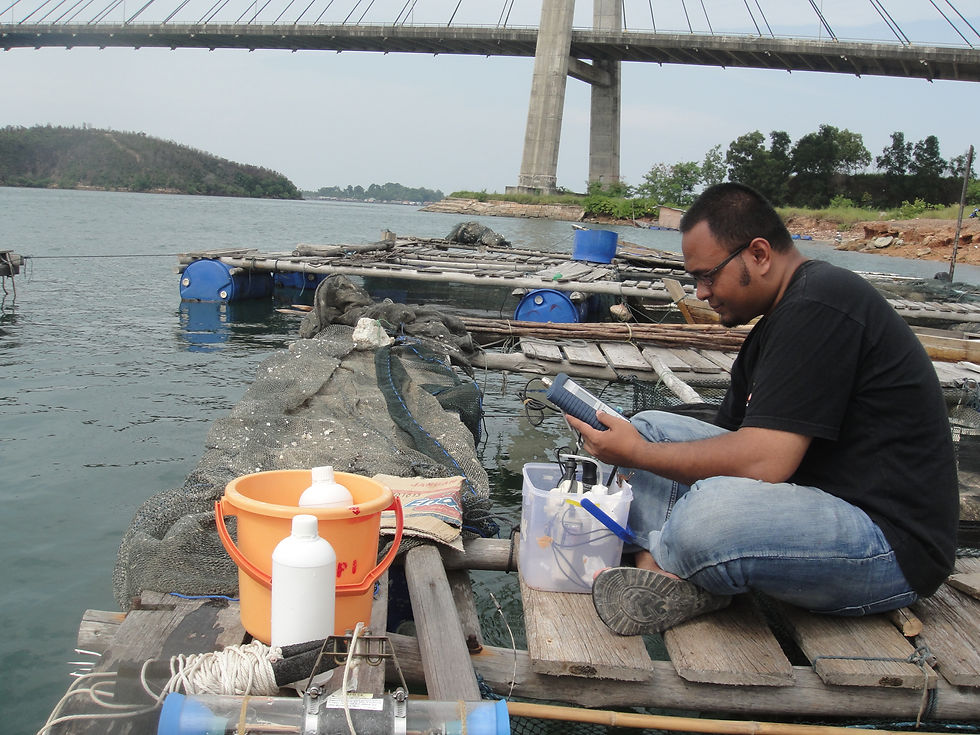
Mariculture is one category of aquaculture production involving the cultivation of marine organisms in the sea and intertidal zones as well as those operated with land-based (onshore) production facilities and structures (FAO, 2014). The demand of omnivorous and carnivorous species farmed and harvested in marine and brackish coastal environment, particularly groupers (Epinephelus spp), Atlantic salmon (Salmo salar), European seabass (Dicentrarchus labrax), and seabream (Pagrus major) have grown markedly in the last two decades (Sadovy, 2000; Toranzo et al., 2005). However, the rapid expansion of the mariculture industry also effect the environmental quality due to the use of fish meal or trash fish and other supplemental substances in their diets. Therefore, reducing negative environmental impacts is a key issue for ensuring long-term sustainability and the health system of mariculture production (Troell et al. 2003).
The environmental impact of mariculture production largely depends on culture method, stocking density, feed type, and the application of feeding strategies (Wu, 1995). About 57% of feed N and 76% of feed P in the Norwegian salmonid industry may be released into the environment (Wang et al., 2014). Moreover, in mariculture farming system, about 85% of phosphorus, 80-88% of carbon and 52 – 95% of nitrogen input may be lost into the environment through fed wastage, fish excretion, faeces production, and respiration (Wu, 1995). From this number, some 39% of feed N and 24% of feed P were excreted as dissolved inorganic N (DIN) and dissolved inorganic P (DIP), respectively (Wang et al., 2013). The quality and quantity of wastes depend on feed quality, cultured species, feed management practices and culture system characteristics (Iwama, 1991). In general, most of the nutrients released to the environment is in a dissolved form, particular for nitrogen (Neori et al., 2000). Pollution loading of these nutrients may enrich the water coloumn around the cages (Karakassis et al., 2005), algal blooms and coastal eutrophication (Skogen et al., 2009).
Inorganic Nutrients Interaction in Marine Water
The concentration of Inorganic nutrients in water is a static variable and mainly described as the chemical mass (weight) of an element or compound per unit volume at a particular place or time (Wetzel and Likens, 2000). Certain nutrients, such as magnesium and sodium, are relatively conservative in concentration and unaffected by metabolically altered reduction-oxidation conditions of the water. On the other hand, concentration of nitrogen and phosphorus compounds are highly dynamic because they may be utilized, stored, transformed, and excreted rapidly and repeatedly by various aquatic organisms (Wetzel and Likens, 2000). Marine nitrogen (N) cycle in the oceans is driven by complex biogeochemical transformations, including nitrogen fixation, denitrification, assimilation and anaerobic ammonia oxidation, mediated by microorganisms (Zehr and Kudela, 2011). Atmosphere is an essentially unlimited source of nitrogen for N2-fixing microorganisms and composed of approximately 80% N2 gas. Meanwhile, there is no atmospheric source for phosphorus and must be obtained from recycling organic matter (in shallow or deep water) or terrestrial sources (Zehr and Kudela, 2011).
Inorganic Nutrients Interaction with pH and Alkalinity
Theoretically, the term of pH referred to the negative logarithm of the hydrogen ion activity (Boyd, 2000) and alkalinity referred to the buffering capacity of the carbonate system in water (Wetzel and Likens, 2000). Interest in the pH response to inorganic nutrient enrichment in marine aquatic ecosystem began to increase since the ammonia toxicity as one of the inorganic form of nitrogen is related to the pH differences rather than to the comparatively minor influences of salinity and temperature (Eddy, 2005). According to Weiler (1979) and Boyd (2000) ammonia diffusion from water to air will be greatest when pH and the concentration of total ammonia nitrogen were high.
As recently addressed by Spiteri et al. (2008) high pH values affect more than just the N cycle. At pH values >8.5, rates of phosphate desorption increase and the amount of bioavailable phosphorous in the water column during biologically active periods is also increase. Similarly, rates of biogenic silica dissolution also exponentially rise with pH, increasing dissolved silica concentrations in the water column, particularly at the sediment water interface, during bloom periods (Van Cappellen and Qiu 1997). In general, there is a positive feedback between primary productivity and increasing pH, which increases the bioavailability of limiting nutrients (N, P, Si) in these well-mixed systems Coastal Marine Waters (Nixon et al., 2015).
Typically, it was known that seawater is alkaline due to contain with large amounts of dissolved inorganic carbon (Wetzel and Likens, 2000). Nitrification and nitrogen fixation may affect the alkalinity concentration but Cellular fixation of N2 by itself does not change total alkalinity (Wolf-Gladrow et al., 2007). The assimilation of 1 mole of nitrogen (atoms) leads to an increase of alkalinity by 1 mole when nitrate or nitrite is the nitrogen source and the use of ammonia as the nitrogen source decrease the alkalinity by 1 mole. Moreover, there is no change of alkalinity when molecular nitrogen is used as the N source (Goldman and Brewer (1980). In steady state, the denitrification process will balance the input of reactive nitrate by nitrogen fixation (Schlesinger, 1997). However, because of N2 fixation and denitrification occur in different areas of the marine area, these processes will affect the spatial distribution of total alkalinity (Wolf-Gladrow et al., 2007).
Inorganic Nutrients Interaction in Primary Productivity
In the majority of cases, nitrogen and phosphorus limit the primary productivity (Tyrell, 1999), although iron limitation has also been detected (Entsch et al. 1983). Since the biological demands may be higher than the availability of these elements, environmental sources can regulate or limit the aquatic organism’s productivity. Many key variables control these ecosystem variables, and nitrogen (N) cycle is a critical component of the biogeochemical cycles in the context of the productivity in the water coloumn of the ocean (Zehr and Kudela, 2010). Investigation conducted by Vitousek and Howarth (1991) clearly mentioned that N is a central nutrient for terrestrial and aquatic ecosystem and also as the key element in global environmental change. Most marine plankton can utilize ammonium, nitrite, and nitrate as N sources for growth but cannot fix N2 (Baker et al., 2009). However, the fixation of molecular nitrogen (N2) by microflora, such as by Trichodesmium and other N2-fixing cyanobacteria, can serve as a major input of nitrogen to aquatic ecosystem and may also help to meet the N budget requirement (Zehr and Kudela, 2010).
One of the findings of the biogeochemical approach suggest that phosphorus also believed as the primary nutrients limiting biomass accumulation and have an important impact on the species distribution and ecosystem structure (Wetzel and Likens, 2000). This argument largely based on the existence of biological N2 fixation and the availability of approximately 80% of N2 gas in the atmosphere as an essential unlimited source of N for N2-fixing microorganisms. Meanwhile, there is no atmospheric source for P and P must be obtained from organic matter recycling and ultimately dependent on the balance between terrestrial rock weathering and oceanic burial (Zehr and Kudela, 2010). Despite the assumption that P ultimately limits the primary productivity, marine nutrient experiments often result in growth response when N is added (Elser et al., 2000). However, an even greater response in productivity will be obtained when P is added along with N (Smith, 1984).
Alternative strategy for “healthy” function of aquatic ecosystem
The external supplies of inorganic nutrients to aquatic ecosystems will influence the “healthy” function of coastal marine ecosystems and can cause ecological and toxicological effects that are either directly or indirectly related to the proliferation of primary producers (Camargo and Alonso, 2006). In clear waters, increases in nutrient loading with low water turnover rates will lead to (1) phytoplankton blooms (Smith et al., 1999); (2) development of a region of water column anoxia (Tyson, 1997); (3) macroalgal blooms in shallow estuaries (Valiela et al., 1997); and (4) cause an extensive kills of both invertebrates and fishes (Shumway, 1990). Therefore, eutrophication in fact is the most widespread water quality problem in the US and many other nations (Carpenter et al., 1998).
Among the different inorganic nitrogenous compounds (NH4+ , NH3, NO2- , HNO2, NO3- ) that aquatic animals can take up directly from the ambient water, ammonium and nitrate ions are the least toxic, while unionized ammonia is the most toxic compounds (Boyd, 2000). The toxic effect of unionized ammonia on aquatic animals, particularly on fish, include the following: decrease in the ability of aquatic organisms to excrete ammonia; elevation of blood pH; disruption of enzyme systems; gill damage; histological lesions in various internal organs, increased water uptake and oxygen consumption (Boyd, 2000). Meanwhile, The main toxic action of nitrite on fish and crayfish, is due to the conversion of oxygen-carrying pigments to forms that are incapable of carrying oxygen, causing hypoxia and ultimately death (Camargo and Alonso, 2006). However, because of the ameliorating effect of water salinity (sodium, chloride, calcium and other ions), sea water aquatic organisms seem to be more tolerant to the toxicity of inorganic nitrogenous compounds than freshwater animals (Camargo and Alonso, 2006).
Based on monitoring program at marine fish cage sites, Pearson and Black (2001) concluded that discharges from farms, including nitrogen, represent a significant influx of inorganic nutrients to the marine environment. Thus, dilution is not a sufficient strategy to address the issue and recommend that proper siting, adherence to best management practices, improved feed formulation and Integrated mariculture system with the application of extractive organisms were able to removes a significant fraction of inorganic nutrients within the mariculture production (Troell et al., 2003)
Reference
Benitez-Nelson, C.R. 2000. The Biogeochemical Cycling of Phosphorus in Marine Systems. Earth Sci. Rev., 51: 109–135
Boyd, C. E. 2000. Water quality: an introduction. Boston, MA: Kluwer Academic Publishers.
Camargo, J.A. and Alonso, A. (2006). Ecological and toxicological effects of inorganic nitrogen pollution in aquatic ecosystems: A global assessment. Environment International 32: 831–849
Carpenter, S.R., Caraco, N.F., Correll, D.L., Howarth, R.W., Sharpley, A.N., Smith, V.H., 1998. Nonpoint pollution of surface waters with phosphorus and nitrogen. Ecological Applications 8, 559±568.
Chou, R.L., Su, M.S., Chen, H.Y., 2001. Optimal dietary protein and lipid levels for juvenile cobia (Rachycentron canadum). Aquaculture 193: 81–89
Eddy, F. B. 2005, Ammonia in estuaries and effects on fish. Journal of Fish Biology, 67: 1495–1513
Elser, J.J., Sterner, R.W., Gorokhova, E., Fagan, W.F., Markow, T.A. et al. 2000. Biological stoichiometry from genes to ecosystem. Ecol. Lett 3: 540 – 550
Entsch, B., Sim, R.G. and Hatcher, B.G. 1983 Indications from photosynthetic components that iron is a limiting nutrient in primary producers on coral reefs. Mar Biol 73: 17-30.
FAO. 2014. The state of world fisheries and aquaculture: opportunities and challenges. FAO Fisheries and Aquaculture Department. Food and Agriculture Organization of the United Nations. Rome.
Goldman, J.C., Brewer, P.G., 1980. Effect of nitrogen source and growth rate on phytoplankton mediated changes in alkalinity. Limnol. Oceanogr. 25, 352–357.
Iwama, G.K. 1991. Interaction between aquaculture and the environment. Crit. Rev. Environ. Control 21: 177 - 216
Karakassis, I., Pitta P. and Krom, M.D. (2005). Contribution of fish farming to the nutrient loading of the Mediterranean. Sci Mar 69: 313 – 321
López A. J. 1997. Aquafeeds and the environment. In : Tacon A.G.J. (ed.), Basurco B. (ed.). Feeding tomorrow's fish. Zaragoza : CIHEAM, p. 275-289 (Cahiers Options Méditerranéennes; n. 22)
Neori, A., Shpigel, M., Ben-Ezra, D. 2000. Sustainable integrated system for culture of fish, seaweed and abalone. Aquaculture 186: 279 – 291.
Nixon, S., Autumn J. Oczkowski, M. Pilson, L. Fields, C. Oviatt, and C. Hunt. 2015. On the Response of pH to Inorganic Nutrient Enrichment in Well-Mixed Coastal Marine Waters. Estuaries and Coasts. Estuarine Research Federation, Port Republic, MD, 38(1):232-241,
Olsen, Y. 2011. Resources for fish feed in future mariculture. Aquacult Environ Interact 1, 187–200
Pearson, T.H. and K.D. Black. 2001. The environmental impacts of marine fish cage culture. Pages 1-31 in K.D. Black, editor. Environmental Impacts of Aquaculture. CRC Press, Boca Raton, Florida.
Schlesinger, W.H., 1997. Biogeochemistry: An Analysis of Global Change, 2nd ed. Academic Press, San Diego. 588 pp.
Shumway, S.E. 1990. A review of the eects of algal blooms on shell®sh and aquaculture. Journal of the World Aquacultural Society 21: 65-104.
Skogen, M.D., Eknes, M., Asplin, L.C. and sandvik, A.D. (2009). Modelling the environmental effects of fish farming in a Norwegian fjord. Aquaculture 298: 70 – 75
Smith, S.V. 1984. Phosphorus versus nitrogen limitation in the marine environment. Limnol. Oceanography 29: 1149 – 1160.
Spiteri, C., Van Cappellen, P. and Regnier, P. 2008. Surface complexation effects on phosphate adsorption to ferric iron oxyhydroxides along pH and salinity gradients in estuaries and coastal aquifers. Geochimica et Cosmochimica Acta 72: 3431–3445.
Toranzo, A.E., Magarinos, B. and Romalde, J.L. 2005. A review of the main bacterial fish diseases in mariculture. Aquaculture 246: 37 – 61
Troell, M. Halling, C., Neori, A., Chopin, T., Buschmann, A.H., Kautsky, N. and Yarish, C. 2003. Integrated mariculture: asking the right question. Aquaculture 226: 69 – 90
Tyrell, T. 1999. The relative influences of nitrogen and phosphorus on oceanic primary production. Nature 400: 525 – 531
Tyson, R. 1997. ``Dead zone'' in Gulf of Mexico draws federal-state attention. Environmental Science and Technology 31: 454A - 455A
Valiela, I., McClelland, J., Hauxwell, J., Behr, P.J., Hersh, D., Foreman, K., 1997. Macroalgal blooms in shallow estuaries: controls and ecophysiological and ecosystem consequences. Limnology and Oceanography 42, 1105-1118.
Van Cappellen, P. and Qiu, L. 1997. Biogenic silica dissolution in sediments of the Southern Ocean. II. Kinetics. Deep Sea Research II 44: 1129–1149
Van der Heide, T., A.J.P. Smolders, B.G.A. Rijkens, E.H. van Nes, M.M. van Katwijk, and J.G.M. Roelofs. 2008. Toxicity of reduced nitrogen in eelgrass (Zostera marina) is highly dependent on shoot density and pH. Oecologia 158: 411–419
Vitousek, P.M. and Howarth, R.W. 1991. Nitrogen limitation on land and in the sea: How can it occur?. Biogeochem 13: 87-115.
Wang, X., Andressen, K., Handa, A., Jensen, B., Reitan, K. and Olsen, Y. (2013). Chemical composition and release rate of waste discharge from an Atlantic salmon farm with an evaluation of IMTA feasibility. Aquac Environ Interact 4: 147 – 162.
Wang, X., Broch, O.J., Forbord, S., Handa, A., Skjermo, J., Reitan, K.I., Vadstein, O. and Olsen, Y. 2014. Assimilation of inorganic nutrients from salmon (Salmo salar) farming by the macroalgae (Saccharina latissima) in an exposed coastal environment: implications for integrated multi-trophic aquaculture. J. Appl. Phycol 26: 1869 – 1878.
Wetzel, R. G. and G. E. Likens. 2000. Limnological Analyses. 3rd Edition. Springer-Verlag, New York. 429 pp.
Wolf-Gladrow, D. A., R. E. Zeebe, C. Klaas, A. Körtzinger, and A. G. Dickson. 2007. Total alkalinity: The explicit conservative expression and its application to biogeochemical processes, Mar. Chem., 106(1–2): 287–300.
Wu, R.S.S. 1995. The environmental impact of marine fish culture: Towards a sustainable future. Marine Poluution Bulletin 31: 159 – 166
Zehr, J.P. and Kudela, R.M. 2011. Nitrogen cycle of the open ocean: from genes to ecosystems. Annu. Rev. Mar. Sci., 3: 5.1-5.29
Commentaires